MMPB-MS08
Multiscale simulations of biological fluid dynamics
Tuesday, June 15 at 11:30am (PDT)Tuesday, June 15 at 07:30pm (BST)Wednesday, June 16 03:30am (KST)
Organizers:
Matea Santiago (University of California, Merced, United States), Shilpa Khatri (University of California, Merced, United States)
Description:
Biological fluid dynamics encompass a wide range of scales, from the organism to subcellular levels. The role of fluid dynamics in tissue, organ, and organism scales are of particular interest due to their providing high-level understanding of organism movement and physiology. These scales often involve solving systems of linear and non-linear partial-differential equations. Further, these biological applications typically involve complicated boundary conditions where the fluid interacts with an elastic or rigid moving structure, making these problems mathematically challenging. This has led to the development of advanced numerical methods to gain insight into these interesting problems. In this minisymposium, we present what occurs at the tissue and organ scale where there are many interesting and valuable physiological applications where the role of biological fluid dynamics is significant. For instance, modeling different components of the circulatory system can require fluid dynamics at the organ scale, heart, or at the tissue scale, blood vessel, depending on the problem being investigated. Further we will also discuss the fluid dynamics at the organism scale, specifically fluid flows around sessile and motile marine organisms. These applications can provide insight into open questions relating to marine ecology, engineering problems, and mixing dynamics.
Christiana Mavroyiakoumou
(University of Michigan, United States)"Large amplitude flutter of membranes"
Alyssa Taylor
(North Carolina State University, United States)"Fluid dynamics in hypoplastic left heart syndrome patients in supine and upright positions"
Christina Hamlet
(Bucknell University, Department of Mathematics, United States)"Modeling the small-scale ballistics and fluid dynamics of nematocyst firing"
Ebrahim Kolahdouz
(University of North Carolina at Chapel Hill, United States)"Migration and trapping of deformable blood clots using a sharp interface Lagrangian"
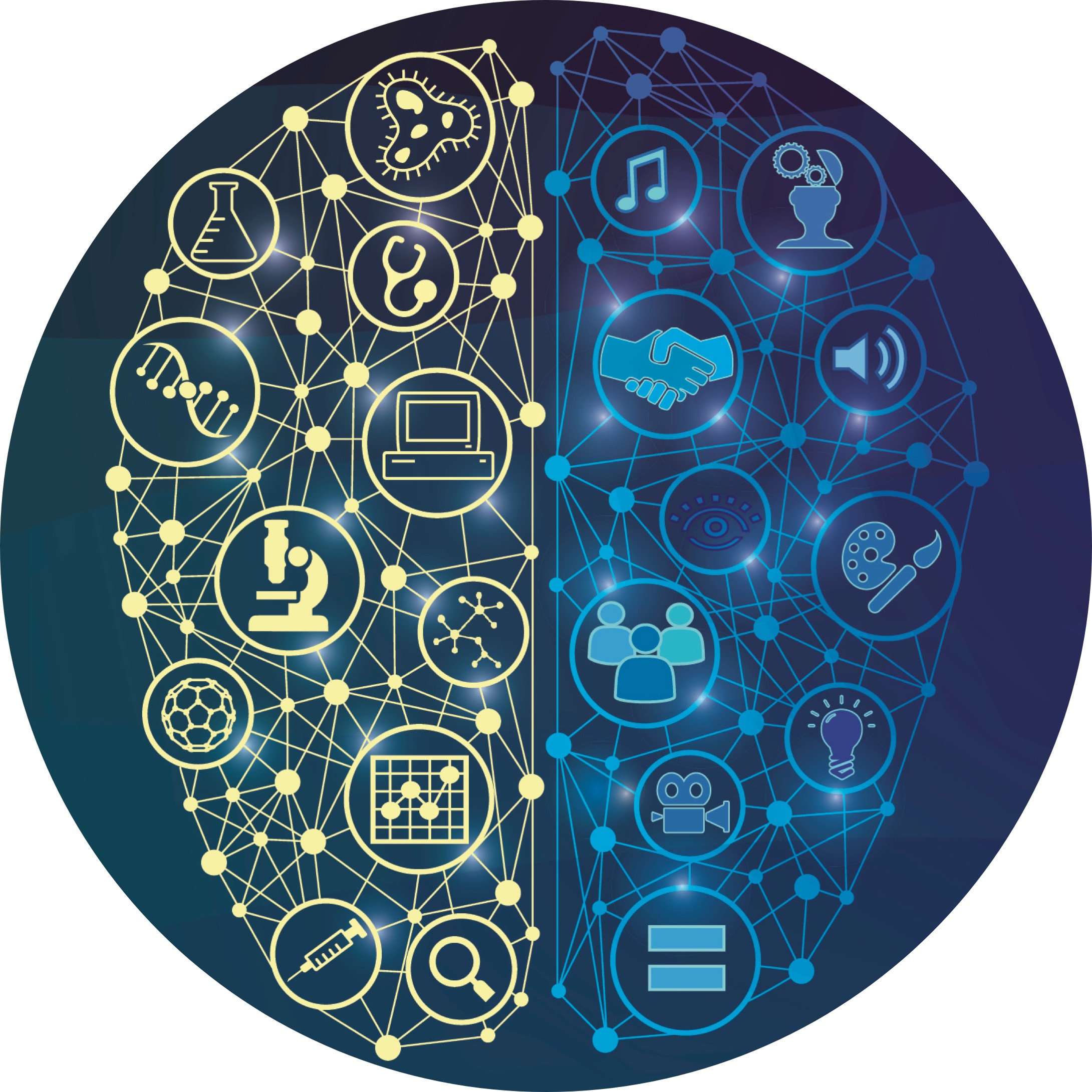