IMMU-MS08
Modeling of lung function and mechanics
Tuesday, June 15 at 11:30am (PDT)Tuesday, June 15 at 07:30pm (BST)Wednesday, June 16 03:30am (KST)
Share this
Organizers:
Jennifer Mueller (Colorado State University, United States)
Description:
While mechanical ventilation is a life-saving technique for patients with respiratory failure, including those with Covid-19 ARDS, it comes with the risk of ventilator-induced lung injury (VILI). This minisymposium focuses on modeling lung function and mechanics, especially in ventilated patients. Both forward and inverse models will be presented, ranging from modeling inflation instability for thick-walled alveoli and modeling of VILI progression to the inverse problems of determining airway resistance along the bronchial tree and diagnosis of VILI from ventilator waveform data.
Bradford Smith
(Department of Bioengineering, University of Colorado Denver | Anschutz Medical Campus, United States)"Ventilator waveform analysis to diagnose and prevent ventilator-induced lung injury"
Emily Heavner
(Colorado State University, United States)"Estimation of airway resistance throughout the bronchial tree from mechanical ventilation output data"
Bela Suki
(Dept. Biomedical Engineering, Boston University, United States)"Inflation instability in the lung: An analytical model of a thick-walled alveolus with wavy fibers under large deformations"
Vitor Mori
(University of Vermont, United States)"Modelling the progression of Ventilation-Induced Lung Injury in Mice"
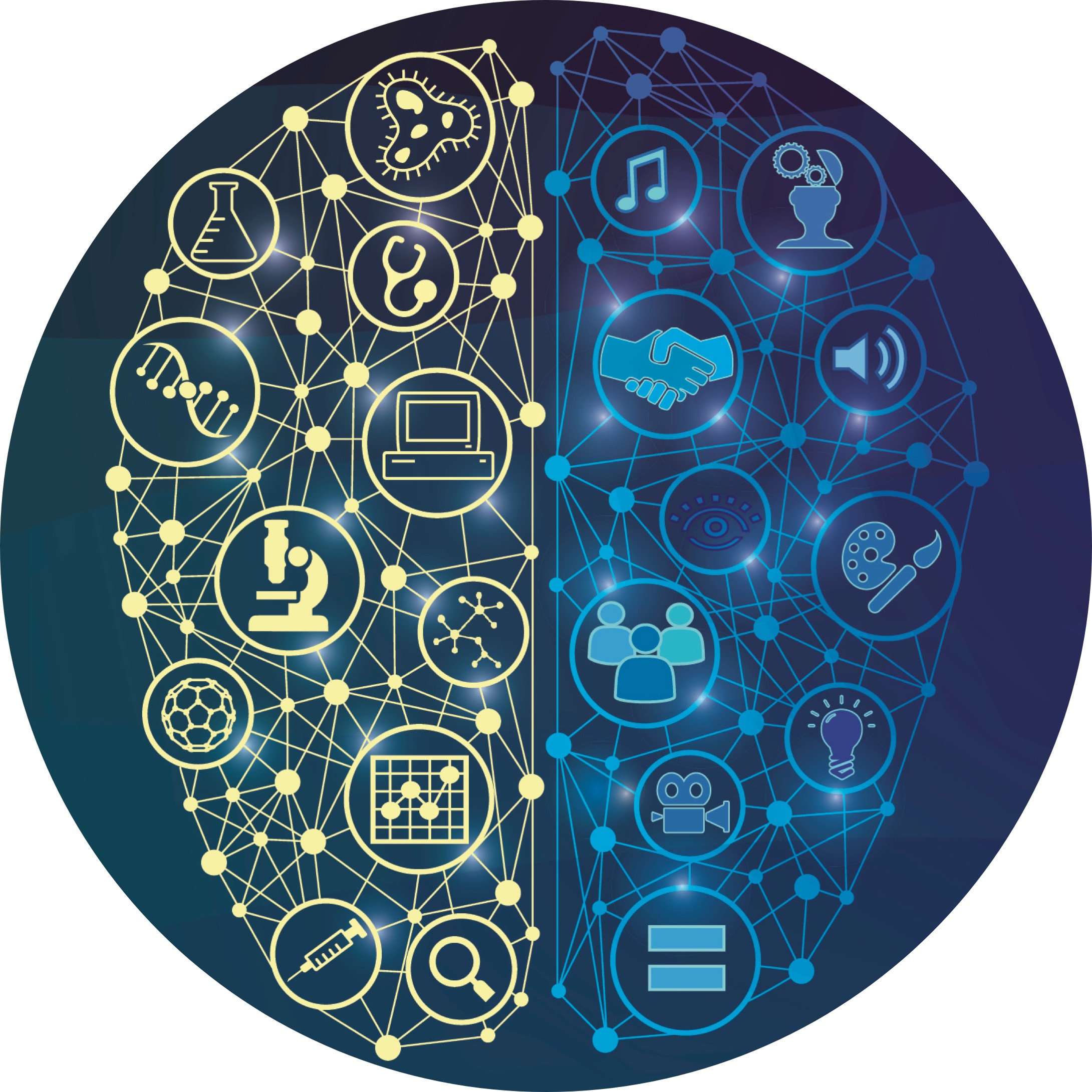