NEUR-MS08
Modeling cardiac electrophysiology and pharmacology in health and disease
Tuesday, June 15 at 11:30am (PDT)Tuesday, June 15 at 07:30pm (BST)Wednesday, June 16 03:30am (KST)
Organizers:
Seth Weinberg (The Ohio State University, United States) & Eleonora Grandi (UC Davis, United States)
Description:
Computational models of cardiac electrophysiology have progressed rapidly in recent years and yielded a vast body of knowledge regarding normal heart physiology and disease mechanisms that would be inimaginable with experimental approaches alone. Models have been successfully applied to understand the mechanisms of cardiac rhythm dysregulation, inform therapeutic anti-arrhythmic strategies, and utilized in defining new predictive paradigms for cardiac safety. These frameworks have become increasingly complex and detailed, e.g., to account for various interacting cell functions and regulation (e.g., by the autonomic nervous system) in (patho)physiological states, and to capture the spatial and temporal details of intracellular and inter-cellular signaling. Although models are generally simplified and computational approaches are not yet truly multiscale with respect to pharmacology, we will present initial efforts to model molecular details of drug-ion channel interaction. While pharmacology applications are still relatively distant from the ultimate goal of model-based personalized treatment, we will discuss novel approaches that use models as a foundation for developing general rules about the interaction of pharmacologic targeting with cardiac dynamic substrate and provide an important intermediate step to the preclinical and clinical pipelines.
Eric Sobie
(Icahn School of Medicine at Mount Sinai, United States)"Creating cell-specific models to infer changes to pig myocyte physiology after myocardial infarction"
Haibo Ni
(University of California Davis, United States)"Quantifying cAMP- and Ca(2+)-dependent proarrhythmic mechanisms using populations of atrial myocyte and tissue models"
Nicolae Moise
(The Ohio State University, United States)"Intercalated Disk Nanoscale Structure Regulates Cardiac Conduction"
Jonathan Silva
(Washington University in St. Louis, United States)"Using molecular detail and genetic background to predict patient response to anti-arrhythmic therapy"
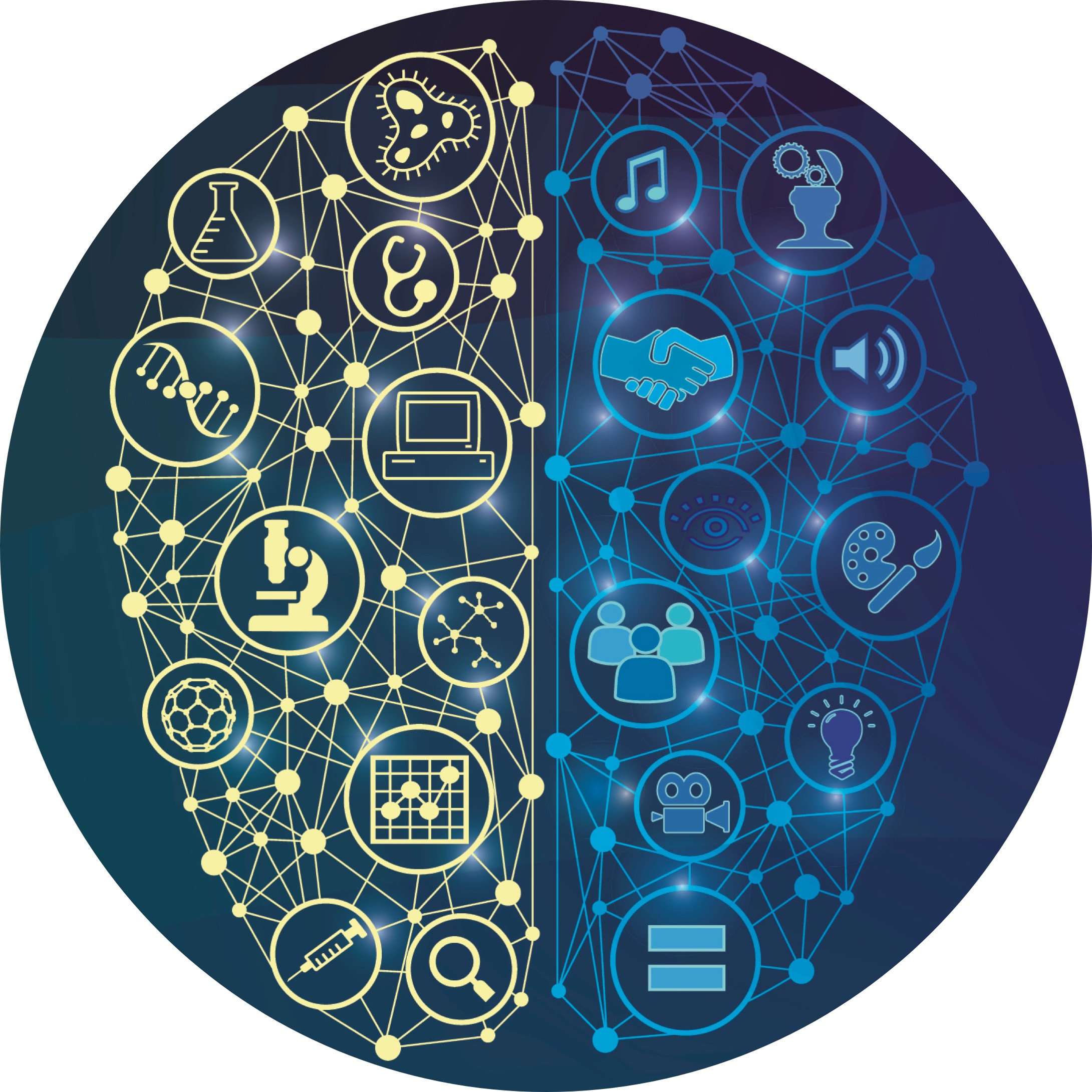