CDEV-MS12
Synergy between experiments and modelling in understanding morphogenetic processes
Wednesday, June 16 at 04:15am (PDT)Wednesday, June 16 at 12:15pm (BST)Wednesday, June 16 08:15pm (KST)
Organizers:
Alessandra Bonfanti (Sainsbury Laboratory University of Cambridge, United Kingdom), Alexandre Kabla (University of Cambridge, United Kingdom)
Description:
Physical forces play a key role in shaping groups of cells into functional tissues. The study of forces during developmental processes started over a century ago with Sir D’Arcy Thompson. Since then, a multidisciplinary approach has been taken up by the scientific community aiming at identifying the physical principles underlying morphogenesis. During the last few years, new breakthroughs have been achieved by combining experimental data with a wide variety of theoretical approaches, from continuum to discrete models, and more recently by integrating artificial Intelligence within the analysis process. In this mini-symposium, we seek to bring together researchers developing advanced quantitative models using different approaches, in close collaboration with leading experimentalists. While providing new insights into developmental processes, the presentations will highlight how the diversity of modelling approaches lead to different interaction and use of experimental data.
Shiladitya Banerjee
(Carnegie Mellon University, USA)"Cell-scale modeling of epithelial morphogenesis using quantitative theory and optogenetics"
Jean-François Rupprecht
(CNRS & Turing Centre for Living Systems Group Leader, Aix-Marseille University., France)"Epithelial tissues flows over hills, valleys and around potholes"
Alan Lowe
(University College London, UK)"Learning the rules of cell competition"
Pasquale Ciarletta
(Politecnico di Milano, Italy)"Pattern formation and self-organization during cancer cell budding in-vitro"
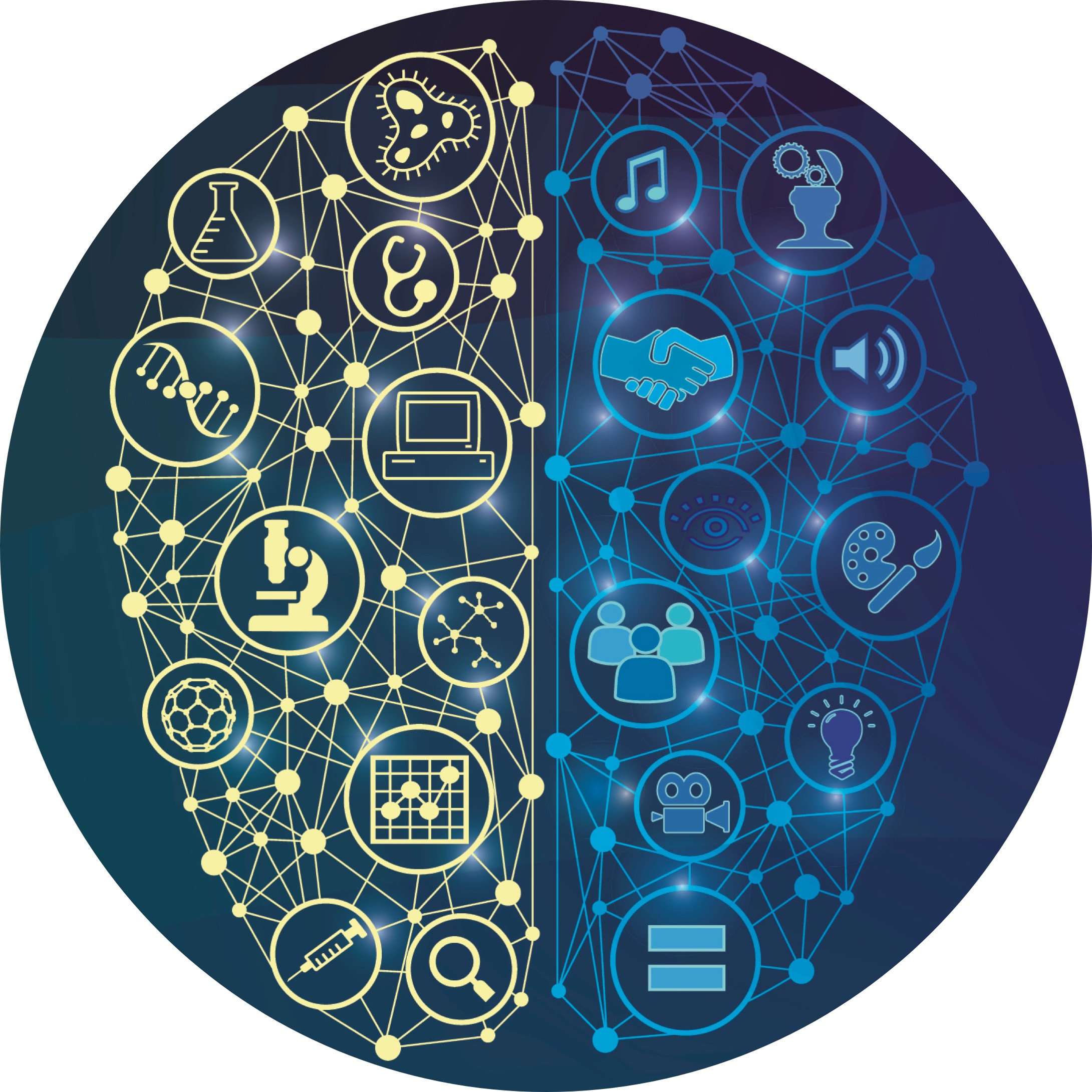