IMMU-MS07
Mathematical and computational virology
Tuesday, June 15 at 09:30am (PDT)Tuesday, June 15 at 05:30pm (BST)Wednesday, June 16 01:30am (KST)
Organizers:
Roya Zandi (University of CA, Riverside, USA), Amber Smith (University of Tennessee, USA), Reidun Twarock (University of York, UK)
Description:
This section will address the modelling of viruses at different scales. At the single particle level, we will focus on geometric models of the protein shell called the capsid, which provides protection and transport for the viral genomes, as well as its assembly. This section will also include the repurposing of viral capsids in nanotechnology and therapy. Multi-scale models of replication dynamics within an infected cell, will cover the processes that allow viruses to take over the cellular machinery of infected cells in order to synthesize the building blocks for their progeny, and track the evolution of the viral quasispecies. Due to the wide spectrum of different processes and complexity levels, collaborative efforts of researchers with a range of expertise are required. This section brings together mathematicians, mathematical physicists, biophysicists and biologists to discuss experimental and theoretical challenges at the forefront of virology research. To foster cross-disciplinary communication and identify complementary areas of expertise, sessions include speakers from different disciplines focusing on similar aspects of virology. Key topics include the understanding of virus architecture and assembly including the use and repurposing of viral capsids in nanotechnology, as well as intra- and intercellular infection models.
Carolyn Teschke
(Department of Molecular and Cell Biology, University of Connecticut, USA)"Using a scaffold to build a viral capsid"
Siyu Li
(Northwestern University, China)"The physical mechanism of virus self-assembly"
Trevor Douglas
(Department of Chemistry, Indiana University, Bloomington IN 47405, USA)"Directed Assembly of Virus-Based Nanoreactors Across Multiple Lengthscales"
Giuliana Indelicato
(Department of Mathematics, The University of York, UK)"The role of surface stress in non-quasi-equivalent viral capsids"
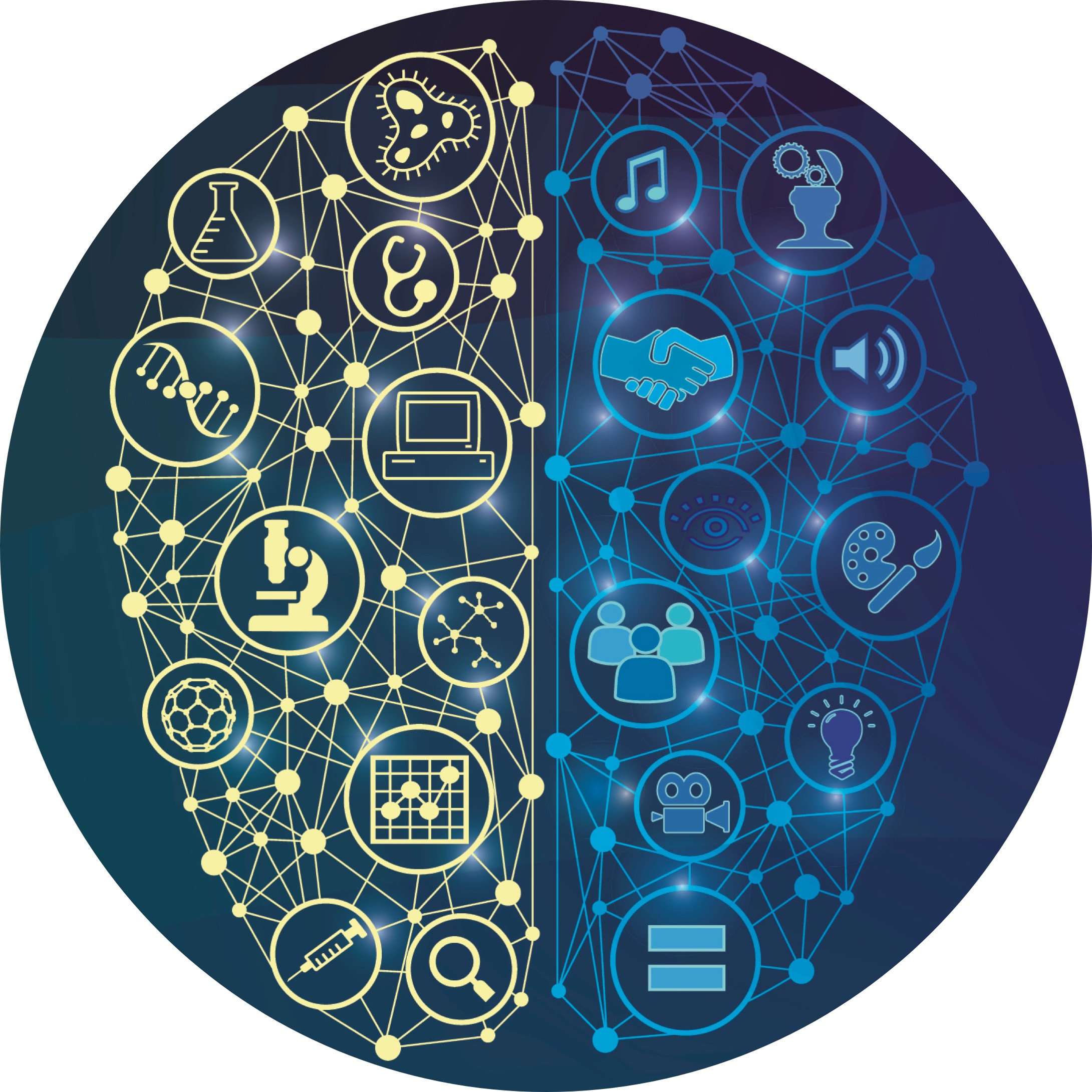