CDEV-MS06
Computational models of extracellular matrix effects on cell migration and tissue formation
Tuesday, June 15 at 04:15am (PDT)Tuesday, June 15 at 12:15pm (BST)Tuesday, June 15 08:15pm (KST)
Organizers:
Magdalena Stolarska (University of St. Thomas, United States), Lisanne Rens (Delft University of Technology, Netherlands)
Description:
During development, health and disease, cells actively migrate as single cells or collectively. Vital processes in which cell migration plays a role include embryogenesis, wound healing and cancer metastasis. Cells and tissues are surrounded by the extracellular matrix (ECM), a network of proteins and fibers. Cells have complex interactions with the ECM, as they deposit matrix fibers, pull on matrix fibers, respond to various cues like fiber alignment and ECM stiffness. To understand how cell migration is guided by the ECM, computational modeling is a powerful tool. It allows us to (more readily, compared to wet-lab experiments) decompose different mechanical aspects, such as ECM stiffness, and study its effect on cell migration. In this field of mathematical biology, many models have been developed. The aim of this mini- symposium is to bring together the diverse modeling approaches of specific ECM cues, and also bridge single cell migration to collective cell behavior and whole tissues. We invite people to talk about their modeling approach and their gained biological insights. Four talks will focus on single cell migration, and four talks will be about collective cell migration and tissue formation. The studied extracellular matrix cues range from ECM density, stiffness, and fiber alignment.
Leonie van Steijn
(Leiden University, Netherlands)"Obstacle-induced contact-inihibition of locomotion explains topotactic cell navigation in dense microenvironments"
Lisanne Rens
(Delft University of Technology, Netherlands)"Computational models for feedback between cell shape, cell signaling and extracellular matrix"
Magda Stolarska
(University of St. Thomas, United States)"Modeling the effects of membrane mechanics on cell-substrate interaction during spreading"
Wanda Strychalski
(Case Western Reserve University, United States)"Computational estimates of mechanical constraints on cell migration through the extracellular matrix"
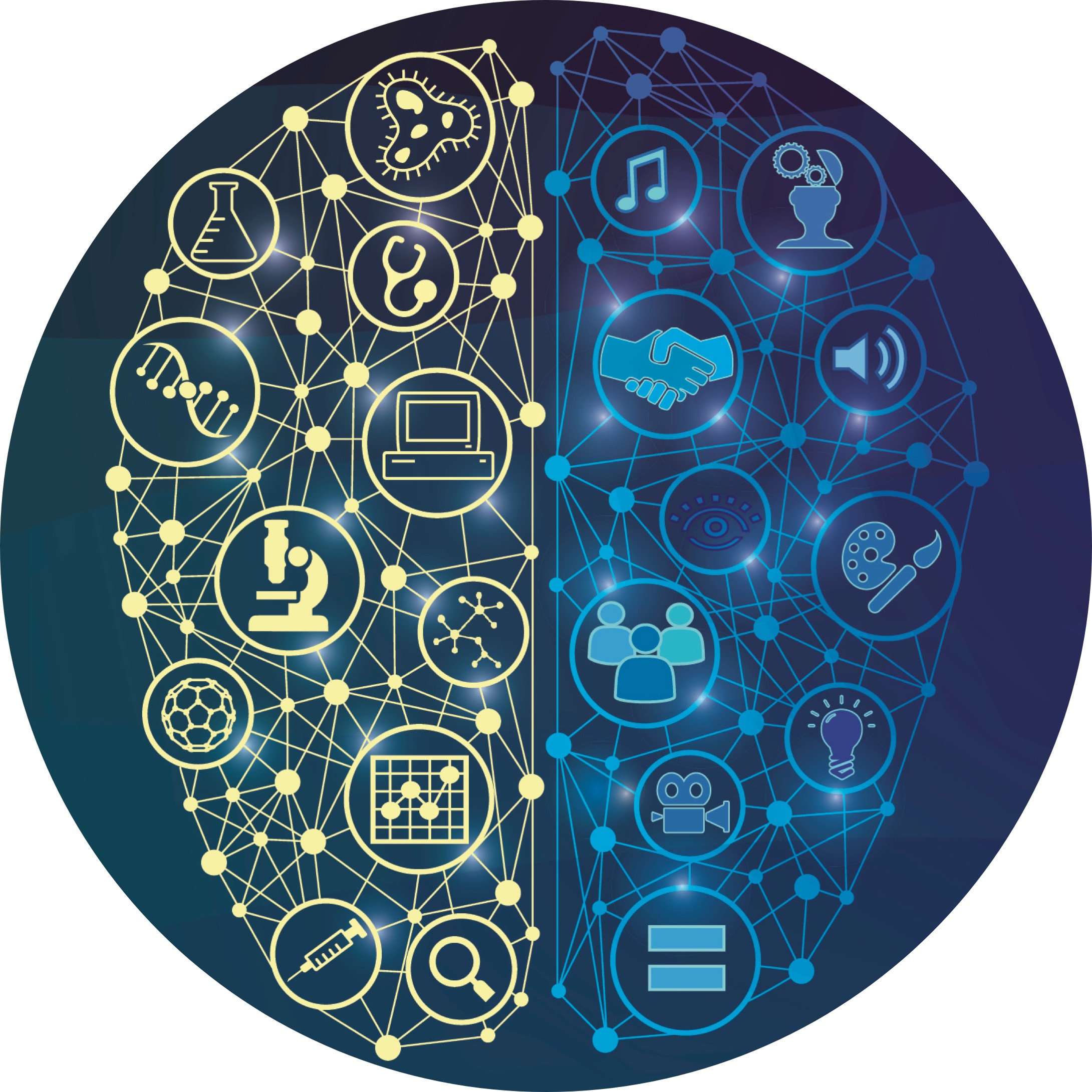