MEPI-MS17
Modelling the transmission of COVID-19 in indoor spaces
Thursday, June 17 at 02:15am (PDT)Thursday, June 17 at 10:15am (BST)Thursday, June 17 06:15pm (KST)
Organizers:
Raquel González Fariña (Cardiff University, United Kingdom), Katerina Kaouri (Cardiff University, United Kingdom)
Description:
It will take time to achieve herd immunity against SARS-CoV-2 through vaccination. In the meantime, we need to find ways to safely lift restrictions and resume economic and social activities while containing the virus. Therefore, indoors transmission of COVID-19 has been intensively researched since the beginning of the pandemic. There is an emphasis on quantifying viral transmission in areas with a high density of people. These high-risk spaces include healthcare clinics, schools, public transport, nursing homes and supermarkets. In this mini-symposium, we present several mathematical approaches for modelling the dynamics of transmission of COVID-19 in diverse indoor locations. All three main routes of transmission – airborne (through droplets and aerosols) and through surfaces (fomite) will be discussed and the effects of ventilation, antiviral technologies, and other non-pharmaceutical interventions will be presented. Deterministic and stochastic mathematical models are considered and are solved using a combination of analytical and numerical techniques. In many cases, Computational Fluid Dynamics simulations are employed to model air flows and transport of SARS-CoV-2 in detail. Many of these results have been used to inform policies related to the COVID-19 pandemic in the UK and elsewhere and the speakers comprise an interdisciplinary group from academic and government organisations.
Christian Kähler
(Universität der Bundeswehr München, Germany)"From droplets to pandemic – how to prevent SARS-CoV-2 infections via droplets and aerosols"
Chenfeng Li
(Swansea University, United Kingdom)"CFD simulation of airborne virus transmission aided by a machine learning surrogate model"
Simon Parker
(Defence Science and Technology Laboratory, United Kingdom)"Transmission of virus in carriages - development of a multiroute viral exposure model for public transport"
Raquel González Fariña
(Cardiff University, United Kingdom)"Predicting the spatiotemporal risk of airborne infection in indoor spaces using an advection-diffusion-reaction equation"
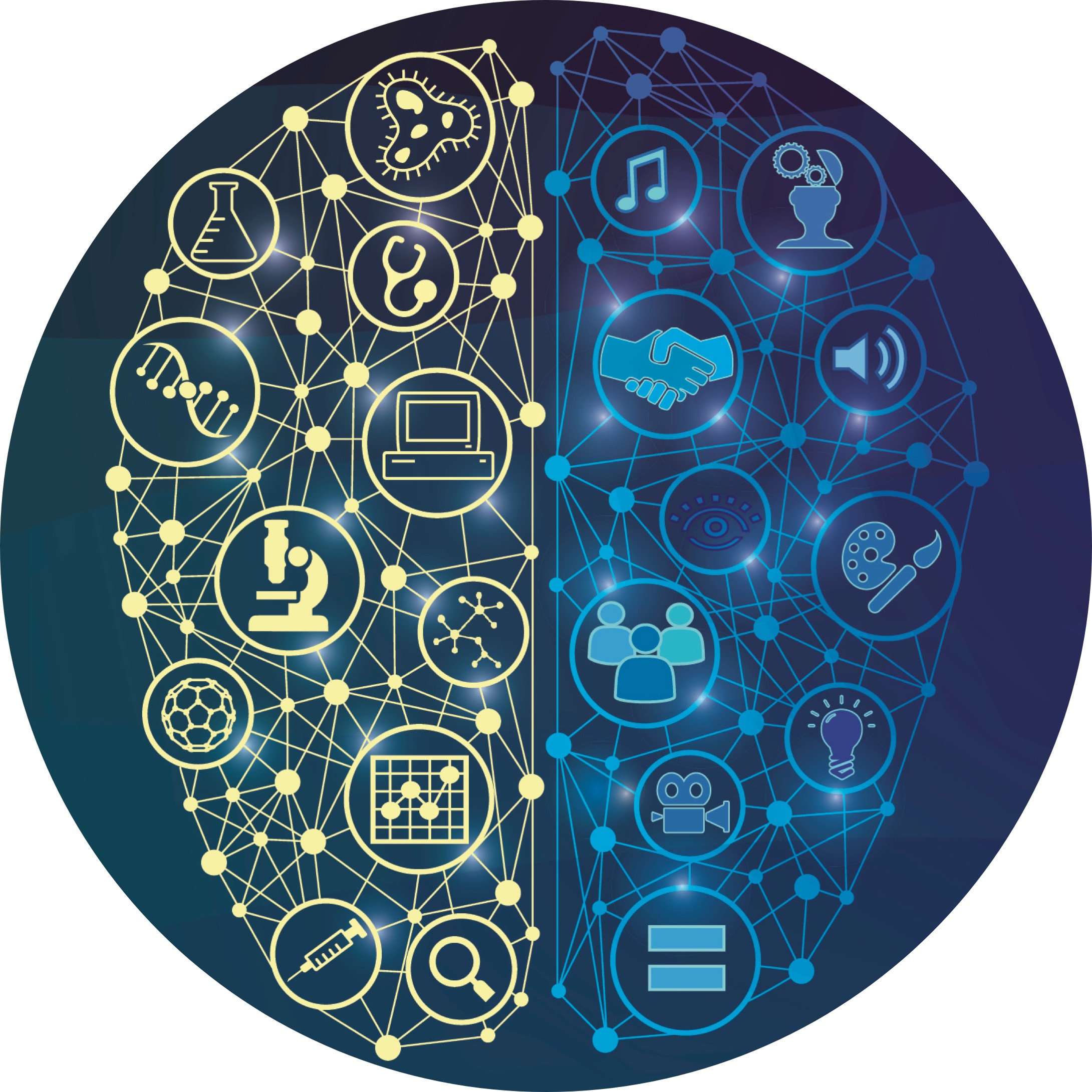